Laboratory of OptoSpintronics
Laboratory of Optospintronics (LOS) is a joint laboratory of Faculty of Mathematics and Physics, Charles University in Prague and Institute of Physics, Academy of Sciences CR. The Laboratory was established in 2011 as an output of an extensive collaboration between Division of Quantum Optics and Optoelectronics Faculty of Mathematics and Physics and Department of Spintronics and Nanoelectronics of the Institute of Physics. The Head of LOS is P. Němec (e-mail: nemec@karlov.mff.cuni.cz).
From 2020, LOS is part of Large Research Infrastructure CzechNanoLab.
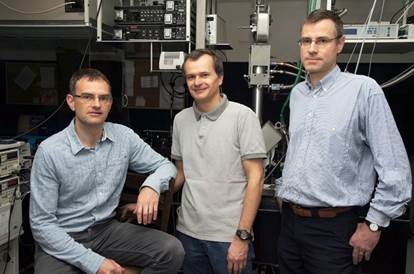
From left to right: Tomáš Jungwirth, Petr Němec, and Vít Novák. Photo by Renata Louvarová.
Optospintronics
Spintronics is a new, dynamically developing branch of electronics that uses the magnetic moment – spin – of charge carriers to store and process data. New technological concepts have been studied in spintronics since the beginning of the eighties, and the first components have already found their commercial application (reading heads for hard disks or magnetic memories of the „MRAM“ type). Recently, light has also started to be used in spintronics, giving rise to its new branch „optospintronics“. Using the interaction of light with spin polarization provides us with new possibilities to non-invasively change and study the magnetic order in a material on very short time scales (up to femtoseconds), which is several orders of magnitude faster than other existing experimental techniques. Our research in the Laboratory of OptoSpintronics (LOS) is mainly focused on the study of mechanisms by which the orientation of magnetic moments in ferromagnetic and antiferromagnetic materials can be changed on ultra-short time scales using laser pulses.
Experimental methods
The impact of a femtosecond excitation pulse on a magnetically ordered material induces changes in the magnetic order. These are subsequently detected by measuring the twist of the polarization plane of the reflected (or emitted) linearly polarized probe pulse as a function of the time delay Δt between the two pulses. The orientation of the magnetization in the sample is described by the polar angle φ and the azimuthal angle θ. An external magnetic field Hext is applied in the plane of the sample at an angle φH .
Materials studied
Time-resolved laser
spectroscopy has been used to study various magnetically ordered materials (both
ferromagnetically and antiferromagnetically) as well as non-magnetic
(paramagnetic) materials.
1) Antiferromagnetic metal CuMnAs
The control and detection of spin ordering in ferromagnets is a fundamental principle currently used to store and read magnetically encoded information. In contrast, the large class of antiferromagnetically ordered materials is much less used, despite the fact that this type of materials has many very attractive properties for spintronic applications. For example, the absence of an external magnetic magnetic moment and scattered magnetic fields eliminates the mutual magnetic interaction between adjacent components. Furthermore, the absence of magnetization in antiferromagnets allows for much faster spin manipulation than is possible with ferromagnets. But the control of spins in antiferromagnets is problematic because it requires very high magnetic fields. Furthermore, it is not possible to use ordinary magnetometers to study the antiferromagnetic arrangement. However, it is possible to use light to study and control the magnetic arrangement in antiferromagnets [see arXiv: 1705.10600].
For this purpose, we have developed an optical method that enables the experimental determination of the direction of the Néel vector in thin metal antiferromagnetic films directly from the measured data and without the need to fit them with a theoretical model [see Nature Photonics 11, 91–97 (2017)]. We demonstrated this experimental method, which is based on a femtosecond excitation and probing experiment using the magneto-optical Voigt effect, in a thin film of the antiferromagnetic metal CuMnAs, which is a very interesting material that was used for the first realization of an antiferromagnetic memory element.
2) Paramagnetic semiconductor GaAs
Long-range and fast transport of spin-polarized charge carriers is one of the key prerequisites for the realization of efficient spintronic logic components. To achieve this, we used the optical excitation of the undoped surface layer at the GaAs/AlGaAs interface, where a conductive layer with a long lifetime is formed, in which the mobility of the charge carriers is not reduced by scattering on the dopants. To demonstrate the unique spin transport properties of this structure, we used a time- and space-resolved method of excitation and probing. These experiments demonstrated that in this structure electron spins can be transported over distances exceeding 10 μm in times less than 1 nanosecond [see Scientific Reports 6, 22901 (2016)].
3) Ferromagnetic semiconductor GaMnAs
Due to the direct band gap in the semiconductor GaAs and the strong exchange interaction between the charge carrier spins and the ferromagnetically ordered Mn ions, (Ga,Mn)As is a particularly suitable material for observing optical spin torques. However, achieving the reproducible growth of (Ga,Mn)As layers and the precise determination of their micromagnetic parameters – such as the Gilbert damping factor or spin stiffness – represents a very difficult goal that we have only recently succeeded in achieving [see Nature Commun. 4, 1422 (2013)].
The study of high-quality (Ga,Mn)As epitaxial layers using high-precision magneto-optical experiments allowed us to identify two new phenomena – „Optical Spin Transfer Torque“ and „Optical Spin-Orbit Torque“ , which open a new way to change the orientation of magnetization at subpicosecond time scale.
„Optical Spin Transfer Torque“ (OSTT) represents a non-relativistic phenomenon, when angular momentum (spin) is transferred from optically injected electrons to magnetization in a ferromagnet. The interconnected precessional dynamics of magnetization and spins of photoinjected charge carriers (electrons) results in a twist on the magnetization that is perpendicular to the plane of the sample and simultaneously to the direction of magnetization, and whose orientation is determined by the helicity of the excitation light.
We monitor the dynamics of magnetization using time-resolved magneto-optical (MO) measurements, which are based on the sensitivity of the polarization of reflected light to the direction of magnetization. The precession of magnetization caused by the OSST phenomenon manifests itself as an oscillating MO signal, in which the phase of the oscillations is determined by the rotation of the circularly polarized excitation pulses. The recorded MO signal can be modeled by the Landau-Lifshitz-Gilbert equation (LLG), which allows to reconstruct the magnetization movement in time and thus to confirm the ultrafast (sub 1 ps) initial flip of the magnetization induced by the laser pulses.
The OSTT phenomenon can also be used to efficiently manipulate magnetic domains and domain walls (DWs), as we showed in Nature Commun. 8, 15226 (2017). If the magnetization in a ferromagnet is oriented perpendicular to its surface, the torsion induced by the OSTT is zero at the perpendicular incidence of circularly polarized light. However, at the interface between two domains with opposite orientation of magnetization (i.e. inside the DW), the magnetization smoothly transitions from one perpendicular orientation to the opposite one and thus also has a non-zero component parallel to the sample surface. At this point, the OSTT acts with a non-zero twist, which leads to the movement of the entire DW, the direction of which is controlled by the rotation of the circular polarization of light. These measurements also showed that the DW can be viewed as a quasi-particle that moves by inertia even after the excitation femtosecond optical pulse has died out.
„Optical Spin-Orbit Torque“ (OSOT) is a relativistic phenomenon that occurs in the absence of an external spin polarizer. OSOT arises only on the basis of spin-orbital interaction acting on non-equilibrium charge carriers (holes), which are excited by laser pulses – of any polarization – into the ferromagnet. The interconnected precessional dynamics leads again to the creation of a twist acting on the magnetization and subsequently to the precession of the magnetization. The main difficulty in this experiment lies in separating the magnetization dynamics originating from the OSOT from the dynamics caused by the temperature mechanism (i.e., laser-induced heating of the sample).
To achieve this goal, we have developed a new experimental technique that allows us to quantitatively reconstruct the 3D trajectory of magnetization in (Ga,Mn)As from magneto-optical (MO) signals, completely without the use of numerical modeling [see Appl. Phys. Lett. 100, 102403 (2012)]. Using this method, we determined the experimental conditions under which the signal associated with OSOT dominates the measured MO data. For example, for low excitation intensities, a slow onset of heat-induced precession prevails, while for higher excitation intensities, there is also an immediate tilting of the magnetization due to OSOT. In addition, the initial tilting of the magnetization due to OSOT can cause precession with such angles that are unavailable in a purely thermal mechanism.